ToF SIMS of Rough or Uneven Samples
A common problem faced by TOF SIMS analysis is loss of peak resolution and mass accuracy on samples with rough or uneven topography. The J105 SIMS does not suffer from these issues, experiencing no loss in mass accuracy across even the roughest of samples
The ability to obtain consistent, accurate results is key to all analytical techniques, and none more so than ToF SIMS. Providing detailed chemical information about a surface, ToF SIMS is an extremely versatile surface analysis technique. The results, however, can be very sensitive to sample environment; insulating samples, or changes in sample height can affect the measurement and result in inconsistent, or inaccurate results.
In this application note we explain the reasons why sample roughness can be a problem, and show how the unique design of the J105 SIMS has solved this issue.
Why Roughness is a Problem for ToF SIMS
Time of flight mass spectrometers determine the mass-to-charge ratio of ions by measuring the time taken to travel a known distance under an electric field. The accuracy of this measurement – known as the mass-resolution – is determined by how precisely this flight time can be measured.
In conventional ToF SIMS, good measurement accuracy is achieved by generating very short pulses (in nanoseconds) of the primary ion beam. If the sample is completely uniform, the variation in flight time of ions with the same mass will be minimal. Thus the experiment will be quite accurate.
Say that our sample is not uniform however, but has a height variation caused by a slight tilt when mounting. In this case, ions of equal mass coming from different parts of the sample will have to travel slightly different paths, and thus will have slightly different flight times. Our experiment will not be as accurate this time, and will show broader peaks as ions of the same mass arrive at slightly different times.
What about a sample with a lot of surface roughness or height variation, such as the surface of a meteorite, or a coronary stent? In these cases it should be clear that accurate results will be difficult to obtain.
There is also a secondary issue caused by samples with rich topography, particularly those that are naturally insulating. Topographic features may result in the build up of localized surface charge. This localized charge distorts the local electric field, and can thus affect the flight path of ions at these locations.
Solving the Problem: Decoupling The Mass Spectrometer
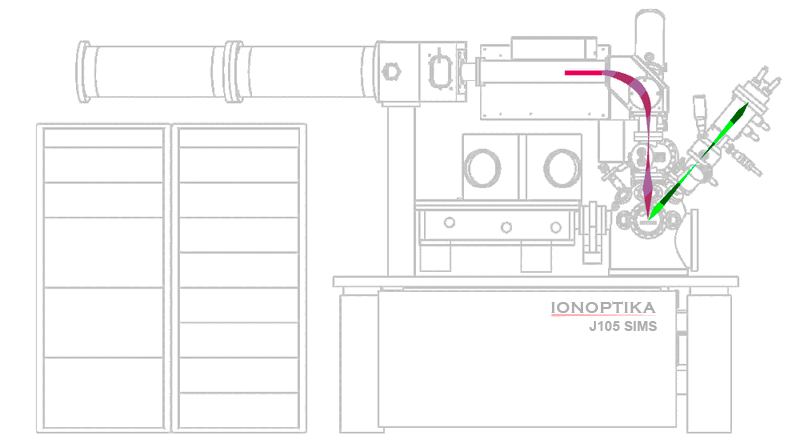
Schematic of the J105 SIMS operation, illustrating the path of primary (green) and secondary (red and blue) ions through the instrument.
In order to solve this problem, the sample and its environment must be decoupled from the mass spectrometer. However, in order to do this, a new method of producing the first time-focus is required.
The J105 SIMS has been designed such that the primary beam is decoupled from the time-of-flight measurement. Instead, a shaped-field buncher and timed ion gate sit after the electrostatic analyzer, generating the first time-focus for the time-of-flight measurement. By cooling the ions prior to passing them into the buncher, the energy spread is reduced to within 1 eV, removing any effects of the sample.
As a result of this design, the distance traveled by the ions prior to reaching the spectrometer has no effect on the measured flight time. The mass spectrometer is therefore independent of the local environment on the sample. This thus enables the J105 to analyze otherwise challenging samples, producing consistent, accurate results with no loss of resolution.
The following examples demonstrate the capabilities of the J105 to analyse rough, uneven, topographic samples without compromising mass resolution or mass accuracy.
Example #1: M1.6 Screw Thread
The figure below shows the total ion image as obtained from an M1.6 screw thread. This sample was chosen for its difficult topography, having a thread depth of approximately 300 μm. Despite the challenging nature of the sample, highly consistent results are obtained no matter what point on the surface is chosen.
The spectrum surrounding the Cr+ peak (nominally 51.94051 amu) is shown to the right of the image for a range of selected areas across the sample. The areas chosen span the full 300 μm depth of the screw thread.
Despite this large variation in height across the sample, the variation in peak center across the chosen points is only 0.0002 amu, which corresponds to a mass error of less than 5 ppm across the complete depth range.
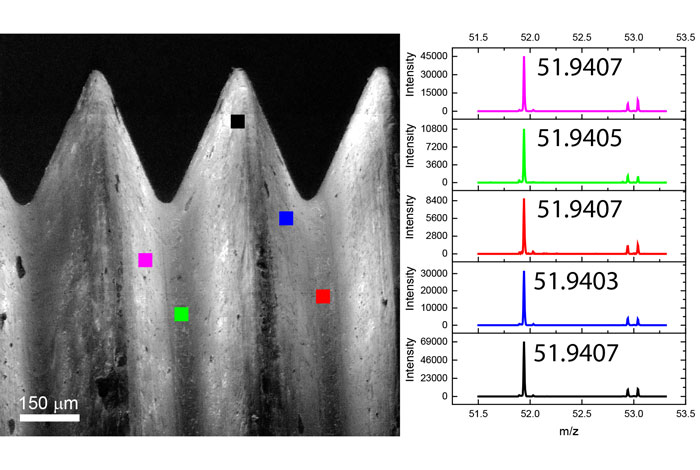
Left Total ion image of an M1.6 screw thread, obtained on the J105 SIMS. Right Comparison of the Cr+ peak (nominal mass 51.94051 amu) from spectra obtained at various locations across the screw thread sample (height variation approximately 300 μm). Variation in mass accuracy across the 300 μm depth range of the sample shows less than 5 ppm deviation from the nominal value.
Example #2: Frog Embryo
The figure below shows the total ion image of a frog embryo during multiple stages of development over a 5.5 hr period. The embryo is a sphere approximately 1.1 mm in diameter – a very challenging samples for ToF SIMS.
Despite the challenging nature of the sample, uniform signal is obtained from almost all areas of the hemisphere without affecting the mass calibration.
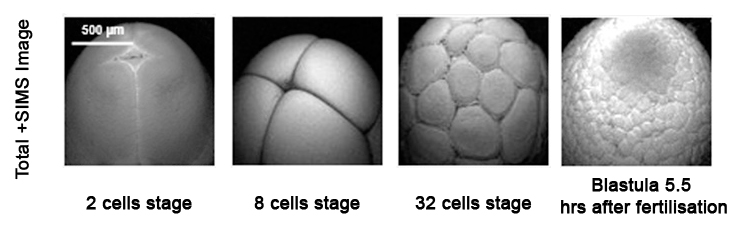
Total ion image of a Xenopus laevis embryo at various stages of development, from 2 cells to many. Embryo is approximately 1.1 mm in diameter, in a field of view of 1200 x 1200 μm. Strong signal is obtained from all parts of the hemisphere, and the mass calibration did not change over the image. This research was originally published in the Journal of Lipid Research. Tian, H. J. Lipid Res. 2014. 55: 1970-1980. Published with permission from original author.
Conclusions
Rough, uneven, or highly topographic samples can cause problems in ToF SIMS analysis, resulting in loss of mass accuracy and mass resolution. Unfortunately, most real world samples tend not to be perfectly uniform.
The J105 SIMS solves this problem by decoupling the mass spectrometer from the primary beam, thereby removing any effects of the sample from the time of flight measurement. The result is a ToF SIMS capable of analyzing rough, topographic samples without compromising mass resolution or mass accuracy.
The J105 SIMS combines flexibility with high performance, delivering unprecedented performance on biological and inorganic samples alike. For more information, visit the J105 product page, read our Application Notes, or Contact Us to discuss your interest further.